Êíèãà: Phantoms in the Brain
CHAPTER 1 The Phantom Within
<<< Íàçàä Preface |
Âïåðåä >>> CHAPTER 2 “Knowing Where to Scratch” |
CHAPTER 1
The Phantom Within
For in and out, above, about, below, ’Tis nothing but a Magic Shadow-show Play’d in a Box whose Candle is the Sun, Round which we Phantom Figures come and go.
I know, my dear Watson, that you share my love of all that is bizarre and outside the conventions and humdrum routines of everyday life.
A man wearing an enormous bejeweled cross dangling on a gold chain sits in my office, telling me about his conversations with God, the “real meaning” of the cosmos and the deeper truth behind all surface appearances.
The universe is suffused with spiritual messages, he says, if you just allow yourself to tune in. I glance at his medical chart, noting that he has suffered from temporal lobe epilepsy since early adolescence, and that is when “God began talking” to him. Do his religious experiences have anything to do with his temporal lobe seizures?
An amateur athlete lost his arm in a motorcycle accident but continues to feel a “phantom arm” with vivid sensations of movement. He can wave the missing arm in midair, “touch” things and even reach out and “grab” a coffee cup. If I pull the cup away from him suddenly, he yelps in pain. “Ouch! I can feel it being wrenched from my fingers”, he says, wincing.
A nurse developed a large blind spot in her field of vision, which is troubling enough. But to her dismay, she often sees cartoon characters cavorting within the blind spot itself. When she looks at me seated across from her, she sees Bugs Bunny in my lap, or Elmer Fudd, or the Road Runner. Or sometimes she sees cartoon versions of real people she’s always known.
A schoolteacher suffered a stroke that paralyzed the left side of her body, but she insists that her left arm is not paralyzed. Once, when I asked her whose arm was lying in the bed next to her, she explained that the limb belonged to her brother.
A librarian from Philadelphia who had a different kind of stroke began to laugh uncontrollably. This went on for a full day, until she literally died laughing.
And then there is Arthur, a young man who sustained a terrible head injury in an automobile crash and soon afterward claimed that his father and mother had been replaced by duplicates who looked exactly like his real parents. He recognized their faces but they seemed odd, unfamiliar. The only way Arthur could make any sense out of the situation was to assume that his parents were impostors.
None of these people is “crazy”; sending them to psychiatrists would be a waste of time. Rather, each of them suffers from damage to a specific part of the brain that leads to bizarre but highly characteristic changes in behavior. They hear voices, feel missing limbs, see things that no one else does, deny the obvious and make wild, extraordinary claims about other people and the world we all live in. Yet for the most part they are lucid, rational and no more insane than you or I.
Although enigmatic disorders like these have intrigued and perplexed physicians throughout history, they are usually chalked up as curiosities — case studies stuffed into a drawer labeled “file and forget”. Most neurologists who treat such patients are not particularly interested in explaining these odd behaviors. Their goal is to alleviate symptoms and to make people well again, not necessarily to dig deeper or to learn how the brain works. Psychiatrists often invent ad hoc theories for curious syndromes, as if a bizarre condition requires an equally bizarre explanation. Odd symptoms are blamed on the patient’s upbringing (bad thoughts from childhood) or even on the patient’s mother (a bad nurturer). Phantoms in the Brain takes the opposite viewpoint. These patients, whose stories you will hear in detail, are our guides into the inner workings of the human brain — yours and mine. Far from being curiosities, these syndromes illustrate fundamental principles of how the normal human mind and brain work, shedding light on the nature of body image, language, laughter, dreams, depression and other hallmarks of human nature. Have you ever wondered why some jokes are funny and others are not, why you make an explosive sound when you laugh, why you are inclined to believe or disbelieve in God, and why you feel erotic sensations when someone sucks your toes? Surprisingly, we can now begin to provide scientific answers to at least some of these questions. Indeed, by studying these patients, we can even address lofty “philosophical” questions about the nature of the self: Why do you endure as one person through space and time, and what brings about the seamless unity of subjective experience? What does it mean to make a choice or to will an action? And more generally, how does the activity of tiny wisps of protoplasm in the brain lead to conscious experience?
Philosophers love to debate questions like these, but it’s only now becoming clear that such issues can be tackled experimentally. By moving these patients out of the clinic and into the laboratory, we can conduct experiments that help reveal the deep architecture of our brains. Indeed, we can pick up where Freud left off, ushering in what might be called an era of experimental epistemology (the study of how the brain represents knowledge and belief) and cognitive neuropsychiatry (the interface between mental and physical disorders of the brain), and start experimenting on belief systems, consciousness, mind-body interactions and other hallmarks of human behavior.
I believe that being a medical scientist is not all that different from being a sleuth. In this book, I’ve attempted to share the sense of mystery that lies at the heart of all scientific pursuits and is especially characteristic of the forays we make in trying to understand our own minds. Each story begins with either an account of a patient displaying seemingly inexplicable symptoms or a broad question about human nature, such as why we laugh or why we are so prone to self-deception. We then go step by step through the same sequence of ideas that I followed in my own mind as I tried to tackle these cases. In some instances, as with phantom limbs, I can claim to have genuinely solved the mystery. In others — as in the chapter on God — the final answer remains elusive, even though we come tantalizingly close. But whether the case is solved or not, I hope to convey the spirit of intellectual adventure that accompanies this pursuit and makes neurology the most fascinating of all disciplines. As Sherlock Holmes told Watson, “The game is afoot!”
Consider the case of Arthur, who thought his parents were impostors. Most physicians would be tempted to conclude that he was just crazy, and, indeed, that is the most common explanation for this type of disorder, found in many textbooks. But, by simply showing him photographs of different people and measuring the extent to which he starts sweating (using a device similar to the lie detector test), I was able to figure out exactly what had gone wrong in his brain (see chapter 9). This is a recurring theme in this book: We begin with a set of symptoms that seem bizarre and incomprehensible and then end up — at least in some cases — with an intellectually satisfying account in terms of the neural circuitry in the patient’s brain. And in doing so, we have often not only discovered something new about how the brain works but simultaneously opened the doors to a whole new direction of research.
•
But before we begin, I think it’s important for you to understand my personal approach to science and why I am drawn to curious cases. When I give talks to lay audiences around the country, one question comes up again and again: “When are you brain scientists ever going to come up with a unified theory for how the mind works? There’s Einstein’s general theory of relativity and Newton’s universal law of gravitation in physics. Why not one for the brain?”
My answer is that we are not yet at the stage where we can formulate grand unified theories of mind and brain. Every science has to go through an initial “experiment” or phenomena-driven stage — in which its practitioners are still discovering the basic laws — before it reaches a more sophisticated theory-driven stage.
Consider the evolution of ideas about electricity and magnetism. Although people had vague notions about lodestones and magnets for centuries and used them both for making compasses, the Victorian physicist Michael Faraday was the first to study magnets systematically. He did two very simple experiments with astonishing results. In one experiment — which any schoolchild can repeat — he simply placed a bar magnet behind a sheet of paper, sprinkled powdered iron filings on the surface of the paper and found that they spontaneously aligned themselves along the magnetic lines of force (this was the very first time anyone had demonstrated the existence of fields in physics). In the second experiment, Faraday moved a bar magnet to and fro in the center of a coil of wire, and, lo and behold, this action produced an electrical current in the wire.
These informal demonstrations — and this book is full of examples of this sort — had deep implications1: They linked magnetism and electricity for the first time. Faraday’s own interpretation of these effects remained qualitative, but his experiments set the stage for James Clerk Maxwell’s famous electromagnetic wave equations several decades later — the mathematical formalisms that form the basis of all modern physics.
My point is simply that neuroscience today is in the Faraday stage, not in the Maxwell stage, and there is no point in trying to jump ahead. I would love to be proved wrong, of course, and there is certainly no harm in trying to construct formal theories about the brain, even if one fails (and there is no shortage of people who are trying). But for me, the best research strategy might be characterized as “tinkering”. Whenever I use this word, many people look rather shocked, as if one couldn’t possibly do sophisticated science by just playing around with ideas and without an overarching theory to guide one’s hunches. But that’s exactly what I mean (although these hunches are far from random; they are always guided by intuition).
I’ve been interested in science as long as I can remember. When I was eight or nine years old, I started collecting fossils and seashells, becoming obsessed with taxonomy and evolution. A little later I set up a small chemistry lab under the stairway in our house and enjoyed watching iron filings “fizz” in hydrochloric acid and listening to the hydrogen “pop” when I set fire to it. (The iron displaced the hydrogen from the hydrochloric acid to form iron chloride and hydrogen.) The idea that you could learn so much from a simple experiment and that everything in the universe is based on such interactions was fascinating. I remember that when a teacher told me about Faraday’s simple experiments, I was intrigued by the notion that you could accomplish so much with so little. These experiences left me with a permanent distaste for fancy equipment and the realization that you don’t necessarily need complicated machines to generate scientific revolutions; all you need are some good hunches.2
Another perverse streak of mine is that I’ve always been drawn to the exception rather than to the rule in every science that I’ve studied. In high school I wondered why iodine is the only element that turns from a solid to a vapor direcdy when heated, without first melting and going through a liquid stage. Why does Saturn have rings and not the other planets? Why does water alone expand when it turns to ice, whereas every other liquid shrinks when it solidifies? Why do some animals not have sex? Why can tadpoles regenerate lost limbs though an adult frog cannot? Is it because the tadpole is younger, or is it because it’s a tadpole? What would happen if you delayed metamorphosis by blocking the action of thyroid hormones (you could put a few drops of thiouracil into the aquarium) so that you ended up with a very old tadpole? Would the geriatric tadpole be able to regenerate a missing limb? (As a schoolboy I made some feeble attempts to answer this, but, to my knowledge, we don’t know the answer even to this day.)3
Of course, looking at such odd cases is not the only way — or even the best way — of doing science; it’s a lot of fun but it’s not everyone’s cup of tea. But it’s an eccentricity that has remained with me since childhood, and fortunately I have been able to turn it into an advantage. Clinical neurology, in particular, is full of such examples that have been ignored by the “establishment” because they don’t really fit received wisdom. I have discovered, to my delight, that many of them are diamonds in the rough.
For example, those who are suspcious of the claims of mind-body medicine should consider multiple personality disorders. Some clinicians say that patients can actually “change” their eye structure when assuming different personas — a nearsighted person becomes farsighted, a blue-eyed person becomes brown-eyed — or that the patient’s blood chemistry changes along with personality (high blood glucose level with one and normal glucose level with another). There are also case descriptions of people’s hair turning white, literally overnight, after a severe psychological shock and of pious nuns’ developing stigmata on their palms in ecstatic union with Jesus. I find it surprising that despite three decades of research, we are not even sure whether these phenomena are real or bogus. Given all the hints that there is something interesting going on, why not examine these claims in greater detail? Are they like alien abduction and spoon bending, or are they genuine anomalies — like X rays or bacterial transformation4 — that may someday drive paradigm shifts and scientific revolutions?
I was personally drawn into medicine, a discipline full of ambiguities, because its Sherlock Holmes style of inquiry greatly appealed to me. Diagnosing a patient’s problem remains as much an art as a science, calling into play powers of observation, reason and all the human senses. I recall one professor, Dr. K.V. Thiruvengadam, instructing us how to identify disease by just smelling the patient — the unmistakable, sweetish nail polish breath of diabetic ketosis; the freshly baked bread odor of typhoid fever; the stale-beer stench of scrofula; the newly plucked chicken feathers aroma of rubella; the foul smell of a lung abscess; and the ammonialike Windex odor of a patient in liver failure. (And today a pediatrician might add the grape juice smell of Pseudomonas infection in children and the sweaty-feet smell of isovaleric acidemia.) Inspect the fingers carefully, Dr. Thiruvengadam told us, because a small change in the angle between the nail bed and the finger can herald the onset of a malignant lung cancer long before more ominous clinical signs emerge. Remarkably, this telltale sign — clubbing — disappears instantly on the operating table as the surgeon removes the cancer, but, even to this day, we have no idea why it occurs.
Another teacher of mine, a professor of neurology, would insist on our diagnosing Parkinson’s disease with our eyes closed — by simply listening to the patients’ footsteps (patients with this disorder have a characteristic shuffling gait). This detectivelike aspect of clinical medicine is a dying art in this age of high-tech medicine, but it planted a seed in my mind. By carefully observing, listening, touching and, yes, even smelling the patient, one can arrive at a reasonable diagnosis and merely use laboratory tests to confirm what is already known. Finally, when studying and treating a patient, it is the physician’s duty always to ask himself, “What does it feel like to be in the patient’s shoes?” “What if I were?” In doing this, I have never ceased to be amazed at the courage and fortitude of many of my patients or by the fact that, ironically, tragedy itself can sometimes enrich a patient’s life and give it new meaning. For this reason, even though many of the clinical tales you will hear are tinged with sadness, equally often they are stories of the triumph of the human spirit over adversity, and there is a strong undercurrent of optimism. For example, one patient I saw — a neurologist from New York — suddenly at the age of sixty started experiencing epileptic seizures arising from his right temporal lobe.
The seizures were alarming, of course, but to his amazement and delight he found himself becoming fascinated by poetry, for the first time in his life. In fact, he began thinking in verse, producing a voluminous outflow of rhyme. He said that such a poetic view gave him a new lease on life, a fresh start just when he was starting to feel a bit jaded. Does it follow from this example that all of us are unfulfilled poets, as many new age gurus and mystics assert? Do we each have an untapped potential for beautiful verse and rhyme hidden in the recesses of our right hemisphere? If so, is there any way we can unleash this latent ability, short of having seizures?
•
Before we meet the patients, crack mysteries and speculate about brain organization, I’d like to take you on a short guided tour of the human brain. These anatomical signposts, which I promise to keep simple, will help you understand the many new explanations for why neurological patients act the way they do.
It’s almost a clich? these days to say that the human brain is the most complexly organized form of matter in the universe, and there is actually some truth to this. If you snip away a section of brain, say, from the convoluted outer layer called the neocortex and peer at it under a microscope, you’ll see that it is composed of neurons or nerve cells — the basic functional units of the nervous system, where information is exchanged. At birth, the typical brain probably contains over one hundred billion neurons, whose number slowly diminishes with age.
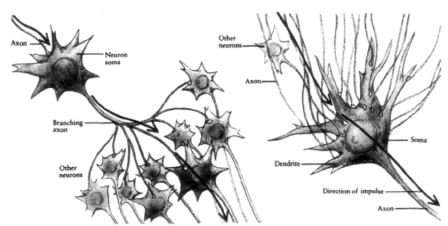
Figure 1.1
Each neuron has a cell body and tens of thousands of tiny branches called dendrites, which receive information from other neurons. Each neuron also has a primary axon (a projection that can travel long distances in the brain) for sending data out of the cell, and axon terminals for communication with other cells.
If you look at Figure 1.1, you’ll notice that neurons make contacts with other neurons, at points called synapses. Each neuron makes anywhere from a thousand to ten thousand synapses with other neurons. These can be either on or off, excitatory or inhibitory. That is, some synapses turn on the juice to fire things up, whereas others release juices that calm everything down, in an ongoing dance of staggering complexity. A piece of your brain the size of a grain of sand would contain one hundred thousand neurons, two million axons and one billion synapses, all “talking to” each other. Given these figures, it’s been calculated that the number of possible brain states — the number of permutations and combinations of activity that are theoretically possible — exceeds the number of elementary particles in the universe. Given this complexity, how do we begin to understand the functions of the brain? Obviously, understanding the structure of the nervous system is vital to understanding its functions5 — and so I will begin with a brief survey of the anatomy of the brain, which, for our purposes here, begins at the top of the spinal cord. This region, called the medulla oblongata, connects the spinal cord to the brain and contains clusters of cells or nuclei that control critical functions like blood pressure, heart rate and breathing. The medulla connects to the pons (a kind of bulge), which sends fibers into the cerebellum, a fist-sized structure at the back of the brain that helps you carry out coordinated movements. Atop these are the two enormous cerebral hemispheres — the famous walnut-shaped halves of the brain. Each half is divided into four lobes — frontal, temporal, parietal and occipital — that you will learn much more about in coming chapters (Figure 1.2).
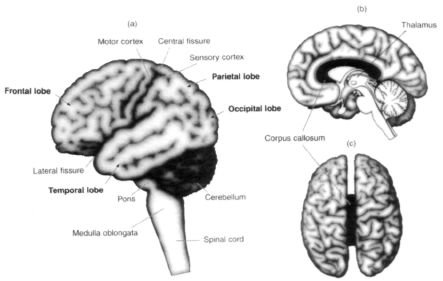
Figure 1.2 Gross anatomy of the human brain, (a) Shows the left side of the left hemisphere. Notice the four lobes: frontal, parietal, temporal and occipital. The frontal is separated from the parietal by the central or rolandic sulcus (furrow or fissure), and the temporal from the parietal by the lateral or sylvian fissure, (b) Shows the inner surface of the left hemisphere. Notice the conspicuous corpus callosum (black) and the thalamus (white) in the middle. The corpus callosum bridges the two hemispheres, (c) Shows the two hemispheres of the brain viewed down the top. (a) Ramachandran; (b) and (c) redrawn from Zeki, 1993.
Each hemisphere controls the movements of the muscles (for example, those in your arm and leg) on the opposite side of your body. Your right brain makes your left arm wave and your left brain allows your right leg to kick a ball. The two halves of the brain are connected by a band of fibers called the corpus callosum. When this band is cut, the two sides can no longer communicate; the result is a syndrome that offers insight into the role each side plays in cognition. The outer part of each hemisphere is composed of cerebral cortex: a thin, convoluted sheet of cells, six layers thick, that is scrunched into ridges and furrows like a cauliflower and packed densely inside the skull.
Right in the center of the brain is the thalamus. It is thought to be evolutionarily more primitive than the cerebral cortex and is often described as a “relay station” because all sensory information except smell passes through it before reaching the outer cortical mantle. Interposed between the thalamus and the cortex are more nuclei, called basal ganglia (with names like the putamen and caudate nucleus). Finally, on the floor of the thalamus is the hypothalamus, which seems to be concerned with regulating metabolic functions, hormone production, and various basic drives such as aggression, fear, and sexuality.
These anatomical facts have been known for a long time, but we still have no clear idea of how the brain works.6 Many older theories fall into two warring camps — modularity and holism — and the pendulum has swung back and forth between these two extreme points of view for the last three hundred years. At one end of the spectrum are modularists, who believe that different parts of the brain are highly specialized for mental capacities. Thus there is a module for language, one for memory, one for math ability, one for face recognition and maybe even one for detecting people who cheat. Moreover, they argue, these modules or regions are largely autonomous. Each does its own job, set of computations, or whatever, and then — like a bucket brigade — passes its output to the next module in line, not “talking” much to other regions.
At the other end of the spectrum we have “holism”, a theoretical approach that overlaps with what these days is called “connectionism”. This school of thought argues that the brain functions as a whole and that any one part is as good as any other part. The holistic view is defended by th fact that many areas, especially cortical regions, can be recruited for multiple tasks. Everything is connected to everything else, say the holists, and so the search for distinct modules is a waste of time.
My own work with patients suggests that these two points of view are not mutually exclusive — that the brain is a dynamic structure that employs both “modes” in a marvelously complex interplay. The grandeur of the human potential is visible only when we take all the possibilities into account, resisting the temptation to fall into polarized camps or to ask whether a given function is localized or not localized.7 As we shall see, it’s much more useful to tackle each problem as it comes along and not get hung up taking sides.
Each view in its extreme form is in fact rather absurd. As an analogy, suppose you are watching the program Baywatch on television. Where is Baywatch localized? Is it in the phosphor glowing on the TV screen or in the dancing electrons inside the cathode-ray tube? Is it in the electromagnetic waves being transmitted through air? Or is it on the celluloid film or video tape in the studio from which the show is being transmitted?
Or maybe it’s in the camera that’s looking at the actors in the scene?
Most people recognize right away that this is a meaningless question. You might be tempted to conclude therefore that Baywatch is not localized (there is no Baywatch “module”) in any one place — that it permeates the whole universe — but that, too, is absurd. For we know it is not localized on the moon or in my pet cat or in the chair I’m sitting on (even though some of the electromagnetic waves may reach these locations). Clearly the phosphor, the cathode-ray tube, the electromagnetic waves and the celluloid or tape are all much more directly involved in this scenario we call Baywatch than is the moon, a chair or my cat.
This example illustrates that once you understand what a television program really is, the question “Is it localized or not localized?” recedes into the background, replaced with the question “How does it work?” But it’s also clear that looking at the cathode-ray tube and electron gun may eventually give you hints about how the television set works and picks up the Baywatch program as it is aired, whereas examining the chair you are sitting on never will. So localization is not a bad place to start, so long as we avoid the pitfall of thinking that it holds all the answers.
So it is with many of the currently debated issues concerning brain function. Is language localized? Is color vision? Laughter? Once we understand these functions better, the question of “where” becomes less important than the question of “how”. As it now stands, a wealth of empirical evidence supports the idea that there are indeed specialized parts or modules of the brain for various mental capacities. But the real secret to understanding the brain lies not only in unraveling the structure and function of each module but in discovering how they interact with each other to generate the whole spectrum of abilities that we call human nature.
Here is where the patients with bizarre neurological conditions come into the picture. Like the anomalous behavior of the dog that did not bark when the crime was being committed, providing Sherlock Holmes with a clue as to who might have entered the house on the night of the murder, the odd behavior of these patients can help us solve the mystery of how various parts of the brain create a useful representation of the external world and generate the illusion of a “self” that endures in space and time.
•
To help you get a feel for this way of doing science, consider these colorful cases — and the lessons drawn from them — taken from the older neurological literature.
More than fifty years ago a middle-aged woman walked into the clinic of Kurt Goldstein, a world-renowned neurologist with keen diagnostic skills. The woman appeared normal and conversed fluently; indeed, nothing was obviously wrong with her. But she had one extraordinary complaint — every now and then her left hand would fly up to her throat and try to strangle her. She often had to use her right hand to wrestle the left hand under control, pushing it down to her side — much like Peter Sellers portraying Dr. Strangelove. She sometimes even had to sit on the murderous hand, so intent was it on trying to end her life.
Not surprisingly, the woman’s primary physician decided she was mentally disturbed or hysterical and sent her to several psychiatrists for treatment. When they couldn’t help, she was dispatched to Dr. Goldstein, who had a reputation for diagnosing difficult cases. After Goldstein examined her, he established to his satisfaction that she was not psychotic, mentally disturbed or hysterical. She had no obvious neurological deficits such as paralysis or exaggerated reflexes. But he soon came up with an explanation for her behavior: Like you and me, the woman had two cerebral hemispheres, each of which is specialized for different mental capacities and controls movements on the opposite side of the body. The two hemispheres are connected by a band of fibers called the corpus callosum that allows the two sides to communicate and stay “in sync”. But unlike most of ours, this woman’s right hemisphere (which controlled her left hand) seemed to have some latent suicidal tendencies — a genuine urge to kill herself. Initially these urges may have been held in check by “brakes” — inhibitory messages sent across the corpus callosum from the more rational left hemisphere. But if she had suffered, as Goldstein surmised, damage to the corpus callosum as the result of a stroke, that inhibition would be removed. The right side of her brain and its murderous left hand were now free to attempt to strangle her.
This explanation is not as far-fetched as it seems, since it’s been well known for some time that the right hemisphere tends to be more emotionally volatile than the left. Patients who have a stroke in the left brain are often anxious, depressed or worried about their prospects for recovery. The reason seems to be that with the left brain injured, their right brain takes over and frets about everything. In contrast, people who suffer damage to the right hemisphere tend to be blissfully indifferent to their own predicament. The left hemisphere just doesn’t get all that upset. (More on this in Chapter 7.)
When Goldstein arrived at his diagnosis, it must have seemed like science fiction. But not long after that office visit, the woman died suddenly, probably from a second stroke (no, not from strangling herself). An autopsy confirmed Goldstein’s suspicions: Prior to her Strangelovean behavior, she had suffered a massive stroke in her corpus callosum, so that the left side of her brain could not “talk to” nor exert its usual control over the right side. Goldstein had unmasked the dual nature of brain function, showing that the two hemispheres are indeed specialized for different tasks.
Consider next the simple act of smiling, something we all do every day in social situations. You see a good friend and you grin. But what happens when that friend aims a camera at your face and asks you to smile on command? Instead of a natural expression, you produce a hideous grimace. Paradoxically, an act that you perform effortlessly dozens of times each day becomes extraordinarily difficult to perform when someone simply asks you to do it. You might think it’s because of embarrassment. But that can’t be the answer because if you walk over to any mirror and try smiling, I assure you that the same grimace will appear.
The reason these two kinds of smiles differ is that different brain regions handle them, and only one of them contains a specialized “smile circuit”. A spontaneous smile is produced by the basal ganglia, clusters of cells found between the brain’s higher cortex (where thinking and planning take place) and the evolutionarily older thalamus. When you encounter a friendly face, the visual message from that face eventually reaches the brain’s emotional center or limbic system and is subsequently relayed to the basal ganglia, which orchestrate the sequences of facial muscle activity needed for producing a natural smile. When this circuit is activated, your smile is genuine. The entire cascade of events, once set in motion, happens in a fraction of a second without the thinking parts of your cortex ever being involved.
But what happens when someone asks you to smile while taking your photograph? The verbal instruction from the photographer is received and understood by the higher thinking centers in the brain, including the auditory cortex and language centers. From there it is relayed to the motor cortex in the front of the brain, which specializes in producing voluntary skilled movements, like playing a piano or combing your hair.
Despite its apparent simplicity, smiling involves the careful orchestration of dozens of tiny muscles in the appropriate sequence. As far as the motor cortex (which is not specialized for generating natural smiles) is concerned, this is as complex a feat as playing Rachmaninoff though it never had lessons, and therefore it fails utterly. Your smile is forced, tight, unnatural.
Evidence for two different “smile circuits” comes from brain-damaged patients. When a person suffers a stroke in the right motor cortex — the specialized brain region that helps orchestrate complex movements on the left side of the body — problems crop up on the left. Asked to smile, the patient produces that forced, unnatural grin, but now it’s even more hideous; it’s a half smile on the right side of the face alone. But when this same patient sees a beloved friend or relative walk through the door, her face erupts into a broad, natural smile using both sides of the mouth and face. The reason is that her basal ganglia have not been damaged by the stroke, so the special circuit for making symmetrical smiles is intact.8
Very rarely, one encounters a patient who has apparently had a small stroke, which neither he nor anyone else notices until he tries to smile. All of a sudden, his loved ones are astonished to see that only one half of his face is grinning. And yet when the neurologist instructs him to smile, he produces a symmetrical, albeit unnatural grin — the exact converse of the previous patient. This fellow, it turns out, had a tiny stroke that only affected his basal ganglia selectively on one side of the brain.
Yawning provides further proof for specialized circuitry. As noted, many stroke victims are paralyzed on the right or left side of their bodies, depending on where the brain injury occurs. Voluntary movements on the opposite side are permanently gone. And yet when such a patient yawns, he stretches out both arms spontaneously. Much to his amazement, his paralyzed arm suddenly springs to life! It does so because a different brain pathway controls the arm movement during the yawn — a pathway closely linked to the respiratory centers in the brain stem.
Sometimes a tiny brain lesion — damage to a mere speck of cells among billions — can produce far-reaching problems that seem grossly out of proportion to the size of the injury. For example, you may think that memory involves the entire brain. When I say the word “rose”, it evokes all sorts of associations: perhaps images of a rose garden, the first time someone ever gave you a rose, the smell, the softness of petals, a person named Rose and so on. Even the simple concept of “rose” has many rich associations, suggesting that the whole brain must surely be involved in laying down every memory trace.
But the unfortunate story of a patient known as H.M. suggests otherwise.9 Because H.M. suffered from a particularly intractable form of epilepsy, his doctors decided to remove “sick” tissue from both sides of his brain, including two tiny seahorse-shaped structures (one on each side) called the hippocampus, a structure that controls the laying down of new memories. We only know this because after the surgery, H.M. could no longer form new memories, yet he could recall everything that happened before the operation. Doctors now treat the hippocampus with greater respect and would never knowingly remove it from both sides of the brain (Figure 1.3).
Although I have never worked directly with H.M., I have often seen patients with similar forms of amnesia resulting from chronic alcoholism or hypoxia (oxygen starvation in the brain following surgery). Talking to them is an uncanny experience. For example, when I greet the patient, he seems intelligent and articulate, talks normally and may even discuss philosophy with me. If I ask him to add or subtract, he can do so without trouble. He’s not emotionally or psychologically disturbed and can discuss his family and their various activities with ease.
Then I excuse myself to go to the restroom. When I come back, there is not a glimmer of recognition, no hint that he’s ever seen me before in his life.
“Do you remember who I am?”
“No.”
I show him a pen. “What is this?”
“A fountain pen.”
“What color is it?”
“It’s red.”
I put the pen under a pillow on a nearby chair and ask him, “What did I just do?”
He answers promptly, “You put the pen under that pillow.”
Then I chat some more, perhaps asking about his family. One minute goes by and I ask, “I just showed you something. Do you remember what it was?”
He looks puzzled. “No.”
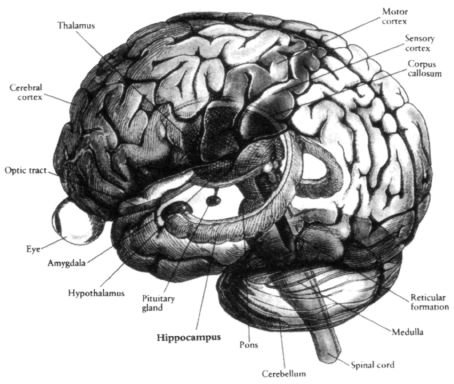
Figure 1.3 Artist’s rendering of a brain with the outer convoluted cortex rendered partially transparent to allow inner structures to be seen. The thalamus (dark) can be seen in the middle, and interposed between it and the cortex are clusters of cells called the basal ganglia (not shown). Embedded in the front part of the temporal lobe you can see the dark, almond-shaped amygdala, the “gateway” to the limbic system. In the temporal lobe you can also see the hippocampus (concerned with memory). In addition to the amygdala, other parts of the limbic system such as the hypothalamus (below the thalamus) can be seen. The limbic pathways mediate emotional arousal. The hemispheres are attached to the spinal cord by the brain stem (consisting of medulla, pons and midbrain), and below the occipital lobes is the cerebellum, concerned mainly with coordination of movements and timing. From Brain, Mind and Behavior by Bloom and Laserson (1988) by Educational Broadcasting Corporation. Used with permission from W. H. Freeman and Company.
“Do you remember that I showed you an object? Do you remember where I put it?”
“No.” He has absolutely no recollection of my hiding the pen sixty seconds earlier.
Such patients are, in effect, frozen in time in the sense they remember only events that took place before the accident that injured them neurologically. They may recall their first baseball game, first date and college graduation in elaborate detail, but nothing after the injury seems to be recorded. For example, if post accident they come upon last week’s newspaper, they read it every day as if it were a brand-new paper each time. They can read a detective novel again and again, each time enjoying the plot and the surprise ending. I can tell them the same joke half a dozen times and each time I come to the punch line, they laugh heartily (actually, my graduate students do this too).
These patients are telling us something very important — that a tiny brain structure called the hippocampus is absolutely vital for laying down new memory traces in the brain (even though the actual memory traces are not stored in the hippocampus). They illustrate the power of the modular approach: In helping to narrow the scope of inquiry, if you want to understand memory, look at the hippocampus. And yet, as we shall see, studying the hippocampus alone will never explain all aspects of memory. To understand how memories are retrieved at a moment’s notice, how they are edited, pigeonholed (sometimes even censored!), we need to look at how the hippocampus interacts with other brain structures such as the frontal lobes, the limbic system (concerned with emotions) and the structures in the brain stem (which allow you to attend selectively to specific memories).
The role of the hippocampus in forming memories is clearly established, but are there brain regions specialized in more esoteric abilities like the “number sense” that is unique to humans? Not long ago I met a gentleman, Bill Marshall, who had suffered a stroke a week earlier. Cheerful and on his way to recovery, he was only too happy to discuss his life and medical condition. When I asked him to tell me about his family, he named each of his children, listed their occupations and gave many details about his grandchildren. He was fluent, intelligent and articulate — and not everyone is so soon after a stroke.
“What was your occupation?” I asked Bill.
Bill replied, “I used to be an Air Force pilot.”
“What kind of plane did you fly?”
He named the plane and said, “It was the fastest man-made thing on this planet at that time.” Then he told me how fast it flew and said that it had been made before the introduction of jet engines.
At one point I said, “Okay, Bill, can you subtract seven from one hundred? What’s one hundred minus seven?”
He said, “Oh. One hundred minus seven?”
“Yeah.”
“Hmmm, one hundred minus seven.”
“Yes, one hundred minus seven.”
“So”, said Bill. “One hundred. You want me to take away seven from one hundred. One hundred minus seven.”
“Yes.”
“Ninety six?”
“No.”
“Oh”, he said.
“Let’s try something else. What’s seventeen minus three?”
“Seventeen minus three? You know I’m not very good at this kind of thing”, said Bill.
“Bill”, I said, “is the answer going to be a smaller number or a bigger number?”
“Oh, a smaller number”, he said, showing that he knew what subtraction is.
“Okay, so what’s seventeen minus three?”
“Is it twelve?” he said at last.
I started wondering whether Bill had a problem understanding what a number is or the nature of numbers.
Indeed, the question of numbers is old and deep, going back to Pythagoras.
I asked him, “What is infinity?”
“Oh, that’s the largest number there is.”
“Which number is bigger: one hundred and one or ninety-seven?”
He answered immediately: “One hundred and one is larger”.
“Why?”
“Because there are more digits.”
This meant that Bill still understood, at least tacitly, sophisticated numerical concepts like place value. Also, even though he couldn’t subtract three from seventeen, his answer wasn’t completely absurd. He said “twelve”, not seventy-five or two hundred, implying that he was still capable of making ballpark estimates.
Then I decided to tell him a little story: “The other day a man walked into the new dinosaur exhibit hall at the American Museum of Natural History in New York and saw a huge skeleton on display. He wanted to know how old it was, so he went up to an old curator sitting in the corner and said, ‘I say, old chap, how old are these dinosaur bones?’”
“The curator looked at the man and said, ‘Oh they’re sixty million and three years old, sir.’”
“‘Sixty million and three years old? I didn’t know you could get that precise with aging dinosaur bones. What do you mean, sixty million and three years old?’”
“‘Oh, well,’ he said, ‘they gave me this job three years ago and at that time they told me the bones were sixty million years old.’”
Bill laughed out loud at the punch line. Obviously he understood far more about numbers than one might have guessed. It requires a sophisticated mind to understand that joke, given that it involves what philosophers call the “fallacy of misplaced concreteness”.
I turned to Bill and asked, “Well, why do you think that’s funny?”
“Well, you know”, he said, “the level of accuracy is inappropriate”.
Bill understands the joke and the idea of infinity, yet he can’t subtract three from seventeen. Does this mean that each of us has a number center in the region of the left angular gyrus (where Bill’s stroke injury was located) of our brain for adding, subtracting, multiplying and dividing? I think not. But clearly this region — the angular gyrus — is somehow necessary for numerical computational tasks but is not needed for other abilities such as short-term memory, language or humor. Nor, paradoxically, is it needed for understanding the numerical concepts underlying such computations. We do not yet know how this “arithmetic” circuit in the angular gyrus works, but at least we now know where to look.10
Many patients, like Bill, with dyscalculia also have an associated brain disorder called finger agnosia: They can no longer name which finger the neurologist is pointing to or touching. Is it a complete coincidence that both arithmetic operations and finger naming occupy adjacent brain regions, or does it have something to do with the fact that we all learn to count by using our fingers in early childhood? The observation that in some of these patients one function can be retained (naming fingers) while the other (adding and subtracting) is gone doesn’t negate the argument that these two might be closely linked and occupy the same anatomical niche in the brain. It’s possible, for instance, that the two functions are laid down in close proximity and were dependent on each other during the learning phase, but in the adult each function can survive without the other. In other words, a child may need to wiggle his or her fingers subconsciously while counting, whereas you and I may not need to do so.
These historical examples and case studies gleaned from my notes support the view that specialized circuits or modules do exist, and we shall encounter several additional examples in this book. But other equally interesting questions remain and we’ll explore these as well. How do the modules actually work and how do they “talk to” each other to generate conscious experience? To what extent is all this intricate circuitry in the brain innately specified by your genes or to what extent is it acquired gradually as the result of your early experiences, as an infant interacts with the world? (This is the ancient “nature versus nurture” debate, which has been going on for hundreds of years, yet we have barely scratched the surface in formulating an answer.) Even if certain circuits are hard-wired from birth, does it follow that they cannot be altered? How much of the adult brain is modifiable? To find out, let’s meet Tom, one of the first people who helped me explore these larger questions.
<<< Íàçàä Preface |
Âïåðåä >>> CHAPTER 2 “Knowing Where to Scratch” |
- Foreword
- Preface
- CHAPTER 1 The Phantom Within
- CHAPTER 2 “Knowing Where to Scratch”
- CHAPTER 3 Chasing the Phantom
- CHAPTER 4 The Zombie in the Brain
- CHAPTER 5 The Secret Life of James Thurber
- CHAPTER 6 Through the Looking Glass
- CHAPTER 7 The Sound of One Hand Clapping
- CHAPTER 8 “The Unbearable Lightness of Being”
- CHAPTER 9 God and the Limbic System
- CHAPTER 10 The Woman Who Died Laughing
- CHAPTER 11 “You Forgot to Deliver the Twin”
- CHAPTER 12 Do Martians See Red?
- Acknowledgments
- Notes
- Bibliography and Suggested Reading
- Index
- Ñîäåðæàíèå êíèãè
- Ïîïóëÿðíûå ñòðàíèöû
- CHAPTER 1 The Phantom Within
- Australopithecus sediba — àâñòðàëîïèòåê, ïîõîæèé íà ÷åëîâåêà
- Èíäðè áåëîëîáûé (Propithecus diadema)
- P. A. Kosintsev Livestock breeding in the forest-steppe and steppe areas of Western Siberia in the late bronze and iron ...
- Quantitative analysis of animal bones from the cultural layers of ancient settlements Summary
- G. Sh. Asylgaraeva To the question about forms of stockbreeding activity of bulgaro-tatar population (on the example of ...
- Òèï Êðóãëûå ÷åðâè, èëè Íåìàòîäû (Nemathelminthes)
- Òèï Ïëîñêèå ÷åðâè (Plathelminthes, èëè Platodes)
- Òàáëèöà 6. Õàðàêòåðèñòèêà ïîëíîñòüþ ðàñøèôðîâàííûõ ãåíîìîâ ðÿäà ïðî– è ýóêàðèîòè÷åñêèõ îðãàíèçìîâ (ïî B. Alberts et al, ...
- Íèâÿíèê îáûêíîâåííûé, èëè ïîïîâíèê (Leucanthemum vulgare)
- Ëþáêà äâóëèñòíàÿ, èëè íî÷íàÿ ôèàëêà (Platanthera bifolia)
- Ðîä Öèàòåÿ (Cyathea)